PLANTS
Photosynthesis
Where do plants get their food?
In 1649, the Dutch physician Jan-Baptisa van Helmont carried out an experiment to test the idea that plants get their food from the soil. He placed a young willow tree (2.25 kg) into a covered pot containing soil (dry weight 90 kg) and watered it daily. After 5 years, he took out the willow tree, weighed it and found it to be 76.1 kgs. The soil in the container after five years was 89.9 kg. The mass of the wilow tree increased greatly, but there was only a difference of 0.1 kg in the soil. This shows that plants do not get energy from their soil, rather another factor.
​
Plants obtain carbon dioxide and energy from the air and sunlight.
Plants are autotrophs. They take simple substances like water and carbon dioxide and transform them into complex organic molecules such as glucose. These organic molelcules contain energy which heterotrophs use.
​
Below is a table which summarizes basic information that is required to do photosynthesis experiments:

Conditions essential for photosynthesis
- Sunlight
- Carbon dioxide
- Chlorophyll
- Water
- A suitable temperature for the plant
​
***Experiments regarding photosynthesis can be found under the Experiments Page.
​
Photosynthesis depends on enzyme reactions in the chloroplasts. Photosynthesis occurs in two stages. The light independent and the light dependent stages.
​
During the light dependent stage:
- Light energy is absorbed by chlorophyll and then converted into chemical energy.
- Light energy is also used to split water molecules into oxygen and hydrogen atoms. This is called photolysis of water.
​
During the light independent stage:
-The hydrogen produced in photolysis is used to reduce (Redox Reactions MYP Chemistry) carbon dioxide to carbohydrates such as glucose. - The energy required for this process comes from the chemical energy formed during the light dependent stage.
- Enzymes play a part in both the light dependent and light independent stages.
Thus, it can be said that:
​
Photosynthesis is the process in which light energy absorbed by chlorphyll is transformed into chemical enetgy. The chemical energy is used to synthesize carbohydrates from water and carbon dioxide. Water and carbon dioxide are the raw materials for photosynthesis. Oxygen is released during the process.
​
The equation for photosynthesis can be written as:
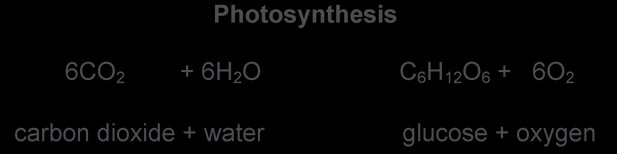
Any factor that directly affects a process if its quantity is changed is called a limiting factor.
Limiting factors of photosynthesis:
- Light intensity
- The concentration of carbon dioxide
- Temperature
​
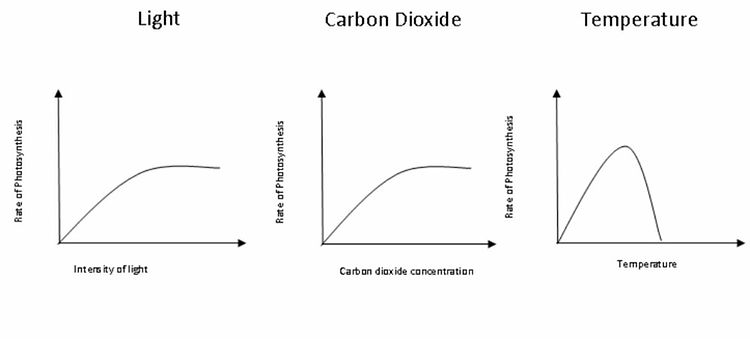
Figure 1. Graphs showing effects of limiting factors on photosynthesis
What happens to glucose that is formed in the leaf?
1. It is used immediately for cellular respiration to provide energy for cellular activities and to form cellulose cell walls.
​
2. (a) In daylight, the rate of photosynthesis is so great that the sugars are formed faster than they can be removed. The excess glucose is converted into starch.
(b) In darkness, photosynthesis stops and starch is converted by enzymes into glucose.
​
3. The glucose in leaves is converted to sucrose which is transported to other parts of the plant or to storage organs (e.g. seeds, stem tubers, or root tubers) via the phloem. After being transported the sucrose is converted into starch or other forms of storage compounds at the storage organs depending on the plant. It might also be converted back into glucose to be used up by the cells.
​
4. Glucose in the leaves can react with nitrates and other mineral salts (absorbed from the soil) to form amino acids in leaves (excess is transported to other parts of plants. Used for synthesis of new protoplasm.) which are then used to form proteins. Proteins are required for the synthesis of new protoplasm.
​
5. Glucose in the leaves also forms fat which is used for storage, cellular respiration and synthesis of new protoplasm.
​
IMPORTANCE OF PHOTOSYNTHESIS
1. Makes chemical energy available to animals and other organisms (introduces energy into food chain)
2. Photosynthesis removes carbon dioxide from the atmosphere and provides oxygen
3. Energy is stored in fossil fuels through photosynthesis (energy captured by plants from the sun, stored in the form of fossil fuels - introduced as complex molecules by plants)
​
​
Leaf Structure & Function
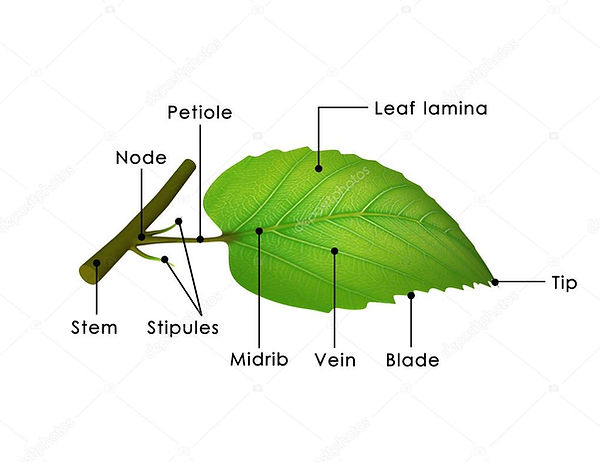

The leaf is the organ where photosynthesis occurs and other food substances like fats and proteins are formed.
​
External Features of a Leaf:
​
Lamina - The lamina has a large flat surface compared to its volume. This enables it to obtain the maximum amount of sunlight for photosynthesis. A large, thin lamina also means that carbon dioxide can rapidly reach the inner cells of the leaf.
​
Network of veins - Veins carry water and mineral salts to the cells in the lamina and carry manufactured food from these cells to other parts of the plant. In a simple leaf, there is a main vein (mid-rib) giving off branches repeatedly, forming a network of fine veins.
​
Leaf arrangement - Leaves are always organized around the stem in a regular pattern. In general, leaves grow either in pairs (opposite one another on the stem) or singly in an alternate arrangement. This ensures that the leaves are not blocking one another from sunlight and that each leaf receives sufficient sunlight.
Petiole - The petiole holds the lamina away from the stem so that the lamina can obtain sufficient sunlight and air. In some leaves, for example grasses and maize, the petiole is absent. Such leaves have long laminae.
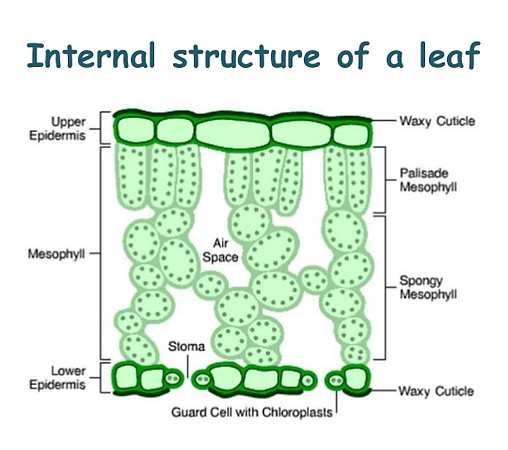
Figure 2. Internal Leaf Structure
Upper Epidermis - The lamina has an upper epidermis made up of a single layer of closely packed cells. The upper epidermis is covered on the outside by a waxy and transparent cuticle.
​
Mesophyll
The mesophyll lies between the upper and lower epidermis. It is the main site of photosynthesis. It consists of two types of tissues:
(a) Palisade Mesophyll - Consists of one or two layers of closely packed, long and cylindrical cells. Cells contain numerous chloroplasts.
(b) Spongy Mesophyll - Cells are irregular in shape. Numerous large intercellular air spaces among the cells present. Cells carry out photosynthesis but contain fewer chloroplasts that the palisade mesophyll cells. Cells are covered with a thin film of moisture. Contains the transport tissues, xylem and phloem. These are grouped to form vascular bundles.
​
Lower Epidermis - Beneath the mesophyll is the lower epidermis. Like the upper epidermis, the lower epidermis consists of a single layer of closely packed cells covered by an outer layer of cuticle which reduces water loss through epidermal cells.
​
Stomata - The lower epidermis contains many minute openings called stomata (sing. stoma)
HOW IS THE LEAF ADAPTED FOR PHOTOSYNTHESIS?
​
Petiole - Holds leaf in position to absorb maximum sunlight
Thin broad lamina - Thin lamina provides a short diffusion distance for gases and enables light to reach all mesophyll cells. Broad lamina provides a large surface area for maximum absorption of light.
Waxy cuticle on upper and lower epidermis - Reduces water loss through evaporation from the leaf; transparent for light to enter the leaf.
Stomata - Open in presence of light, allowing carbon dioxide to diffuse in and oxygen to diffuse out of the leaf.
Chloroplasts containing chlorophyll in mesophyll cells - Chlorophyll absorbs and transforms light energy to chemical energy used in the manufacture of sugars.
More chloroplasts in upper palisade tissue - More light energy can be absorbed near the leaf surface.
Interconnecting system of air spaces in spongy mesophyll - Allows rapid diffusion of CO2 and O2 into and out of mesophyll cells.
Vascular bundle close to mesophyll cells - Xylem transports water and mineral salts to mesophyll cells. Phloem transports sugars away from the leaf.
Guard Cells & Stomata
Stomata generally open in the light and close in the dark.
In sunlight:
1. The guard cells photosynthesize, converting light energy to chemical energy. The chemical energy is used to pump potassium ions into the guard cells from neighbouring epidermal cells.
2. Water potential of the guard cell is lowered. Water from neighboring epidermal cells then enters guard cells by osmosis. This increases turgidity of the guard cells and causes them to become swollen.
3. The guard cells have a thicker cell wall on one side of the cell (the sides around the stomatal pore). This causes the swollen guard cells to become more curved and pull the stomata open.
​
At night:
1. The potassium ions that have accumulated in the guard cells during the day diffuse out of the guard cells.
2. Water potential in the guard cells is increased. Water leaves the cell by osmosis.
3. The guard cells become flaccid and the stomata closes.
​
In this way the guard cells can regulate the rate of diffusion of gases into and out of the leaf, for example, the guard cells can reduce the amount of water vapor escaping from the leaf. Stomata can close to reduce water loss even when a plant is in sunlight, for example, on extremely hot days. In such situations, the excess evaporation of water causes the guard cells to become flaccid. The stomatal pores close and no more water vapor is lost from the plant.
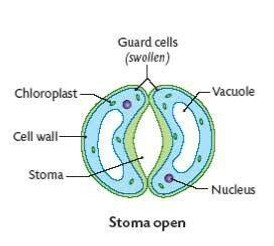

Figure 3. Stomata
HOW DO CARBON DIOXIDE AND WATER ENTER THE LEAF?
​
CO2
1. In daylight when photosynthesis occurs, the carbon dioxide in the leaf is quickly used up. The carbon dioxide concentration in the leaf becomes lower than that in the atmospheric air, so a diffusion gradient exists. Therefore, CO2 diffuses from the surrounding air through the stomata into the air spaces in the leaf.
2. The surfaces of the mesophyll cells are always covered by a thin film of water so that carbon dioxide can dissolve in it.
3. The dissolved carbon dioxide then diffuses into the cells.
​
H2O
1. The xylem transports water and dissolved mineral salts to the leaf from the roots.
2. Once out of the veins, water and mineral salts move from cell to cell right through the mesophyll of the leaf.
​
Transport Structures of Flowering Plants
Flowering plants have a system of vessels that runs up and down the plants carrying materials. These vessels are called transport or vascular tissues. There are two type of transport tissues in plants: the xylem and the phloem.
XYLEM
Structure
- Xylem tissue consists of xylem vessels. A xylem vessel is a long hollow tube stretching from the root to the leaf.
- The xylem vessel is made up of many dead cells.
- The inner walls of the xylem vessels are strengthened with deposits of lignin. Lignin can be deposited in different patterns including ringed and spiral.
Function
- Conducting water and dissolved salts from the roots to the stems and leaves
- Providing mechanical support for the plant.
- The xylem vessel has an empty lumen without protoplasm or 'end-walls'. This reduces resistance to water flowing through the xylem.
- The walls of the xylem are thickened with lignin. This prevents collapse of the vessel and allows the xylem to altogether provide mechanical support to the plant.
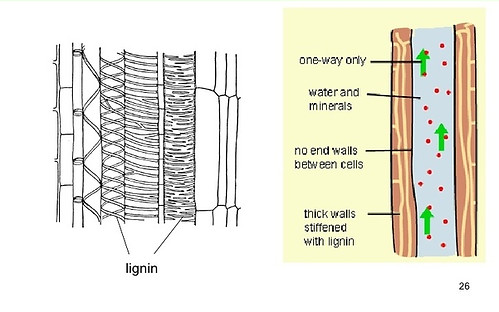
Figure 4. Diagram of xylem

PHLOEM
Structure
- Consists of sieve tubes and companion cells. A sieve tube consists of columns of elongated, thin walled cells called sieve tube cells. The end walls separating the cells have lots of minute pores. The cross walls look like a sieve and are so called sieve plates.
- A mature sieve cell has only a thin layer of cytoplasm inside the cell. This cytoplasm is connected to cells above and below through the sieve plates.
- Each sieve cell has lost its central vacuole, nucleus, and most organelles. Each sieve cell thus has a companion cell beside it which carries out the metabolic processes needed to keep the sieve tube cell alive.
Function
- Conducts manufactured foods (sucrose and amino acids) from the green parts of the plant, to other parts of the plant.
- Companion cells have many mitochondria, which provide energy needed for the companion cell to load sugars from the mesophyll cells into the sieve tubes by active transport.
- The holes in the sieve plates allow rapid flow of manufactured food substances through the sieve tubes.
Figure 5. Diagram of phloem
Arrangement of vascular tissues in stem
1. In a dicotyledonous stem, the xylem and phloem are grouped together to form vascular bundles.
2. The vascular bundles are arranged in a ring around a central region called the pith.
3. The phloem lies outside the xylem with a tissue called the cambium between them. Cambium cells can divide and differentiate to form new xylem and phloem cells, giving rise to a thickening of the stem.
4. The region between the pith and the epidermis is the cortex. Both the cortex and the pith serve to store up food substances, such as starch.
5. The stem is covered by a layer of cells called the epidermis. The epidermal cells are protected by a waxy, waterproof cuticle that greatly reduces evaporation of water from the stem.
​
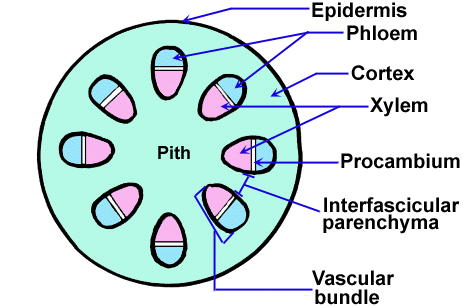
Figure 6. Diagram of the transverse section of a stem

Figure 7. Diagram of the transverse section of a root
Arrangement of vascular tissues in root
1. In a dicotyledonous root, the xylem and phloem are not bundled together, instead, they alternate with each other.
2. The cortex of the root is also a storage tissue.
3. The epidermis of the root is the outermost layer of cells. It bears root hair cells. It is also called the piliferous layer.
4. Each root hair is a tubular outgrowth of an epidermal cell. This outgrowth increases the SA:V of the root hair cell. The absorption of water and mineral salts is increased through this adaptation.
How does water enter a plant?
Each root hair is a fine tubular outgrowth of an epidermal cell. It grows between the soil particles, coming into close contact with the soil solution surrounding them.
The thin film of liquid surrounding each soil particle is a dilute solution of mineral salts.
The sap in the root hair cell is a relatively concentrated solution of sugars and various salts. Thus, the sap has a lower water potential than the soil solution. The water enters through the selectively permeable membrane of the root hair cell from the soil via osmosis.
The entry of water dilutes the sap. The sap of the root hair cell has a higher water potential that that of the next cell. Hence, water passes by osmosis from the root hair cell to the inner cell.
Similarly, water passes from this cell to the next cell of the cortex. This process continues until the water enters the xylem vessels and moves up the plant.
How do root hairs absorb ions or mineral salts?
- By active transport when the concentration of ions in the soil solution is less than that of the root hair cell sap (energy comes from cellular respiration in root hair cell).
​
Root Pressure - When water passes from one cell to the next due to a difference of water potential (as can be seen under the section of 'how does water enter a plant').
Capillary Action - Water tends to move up inside very narrow tubes due to the interaction between water molecules and the surfaces of the tubes. This effect is capillary action.
Transpiration - Transpiration is the combined effect of respiration, evaporation, and photosynthesis, because of which plants lose water vapor from their leaves (the bigger the plant, the more the effect of transpiration).
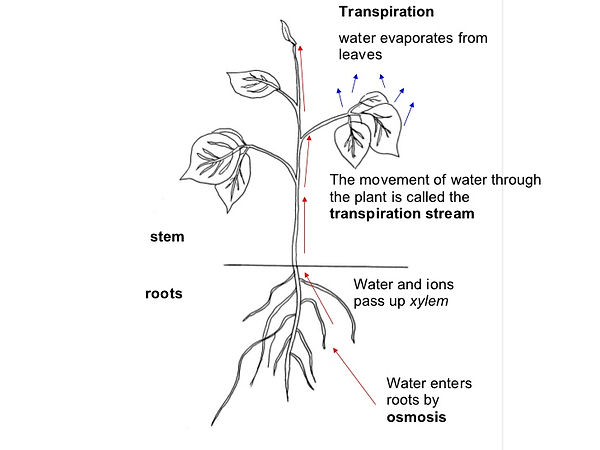
WATER
Adhesive - Sticks to molecules
Cohesive - Stick to other H2O molecules
Figure 8. Effect of Transpiration

Roots
1. Shallow roots - Near surface; straight long branches with few nodes at end; collects run off water and nutrients near the surface.
2. Branching roots - Increase surface area to volume ratio; increase nutrient absorption
3. Dicot tap roots - Deeper into soil to absorb water and mineral ion when minerals start decreasing in soil. Apical meristems present here.
​
IMPORTANCE OF ROOTS
- Anchorage
- Uptake of nutrients, minerals and water
- Maximize SA:V
Roots - Anchorage & water uptake
Stem - Support & transport
Leaves - Gas exchange and photosynthesis
Plant Growth
Storage form of sugar
​
Animals -- Glycogen
Plants -- Starch
Special Plant Features
1. Tendrils - Grow outward from leaves. They are specialized stems which grow and attach to solid surfaces.
2. Bulbs - Specialized thick leaves that store starch.
3. Stem tubers - When food/starch is stored inside roots.
​
(MERISTEMS - Like stem cells)
- Apical Meristems - Make plant grow longitudinally
- Lateral Meristems - Make plant grow sideways
*More nutrients mean apical meristems increase and plant will grow longitudinally.
​
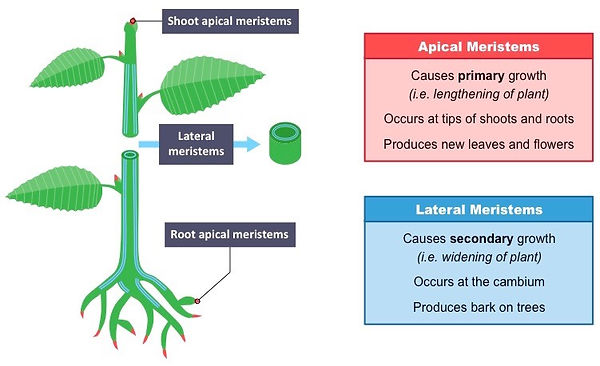
Tropisms - Plants respond to directional stimuli (e.g. they move towards light)
- Negative tropism - Opposite of gravity
- Positive tropism - Towards sunlight
​
*Plant growth is regulated by hormones called auxins.
​
​
Plant Hormones
Auxin - Plant growth
Absisic Acid - Controls rate of transpiration
Figure 9. Location of meristems in plants
MONOCOTS
DICOTS
- Floral organs in multiples of 3s.
- Parallel veins in leaves
- Random distribution of vascular tissue (stem)
- One cotyledon after germination (first leaf)
- Adventitious roots
- Floral organs in multiples of 4s and 5s.
- Branched veins in leaves
- Fixed pattern of vascular tissue (stem)
- Two cotyledons after germination (first leaves)
- Branched roots
Transpiration
Transpiration - Combined effect of respiration, evaporation, and photosynthesis because of which plants lose water vapor from their leaves.
Transpirational pull - Allow water to be moved against the force of gravity.
Transpirational flow is controlled by the rate of water loss through the stomata.
​
Translocation - Movement of food substances in the phloem
​
Rate of Photosynthesis = Rate of Transpiration
​
Factors effecting rate of Transpiration
1. Wind - Rate of transpiration increases because wind blows the water vapor which accumulates outside the stomata away. Helps maintain a constant water vapor concentration gradient between leaf and atmosphere. (The more humid it is, the less the rate of transpiration because less photosynthesis [less of diffusion of gases through stomata])
2. Temperature - A rise in temperature increases the rate of transpiration because it increases the rate of evaporation of water from the cell surfaces.
3. Light - Light increases rate of transpiration because it causes the stomata to open, and thus increase the diffusion of gases, photosynthesis and then transpiration.
4. Humidity - Increasing humidity in the air will result in a decrease in the rate of transpiration because there will be less of a concentration gradient and less diffusion of gases, resulting in a lower rate of photosynthesis.
​
Wilting
The turgor pressure in the leaf mesophyll helps to support the leaf and keep it firm and spread out widely to absorb sunlight for photosynthesis. When the rate of transpiration exceeds the rate of water absorption by the plant, the cells loose their turgor. This causes the cells to become flacid, and the plant wilts.
When leaves wilt, the surface area exposed to sunlight is reduced and the guard cells become flacid (closing stomata), thus lowering the rate of photosynthesis and so the rate of transpiration. Wilting can be advantageous as it makes sure that plants do not lose more water than they have, but it also has disadvantages as it reduces the rate of photosynthesis.
