MOLECULAR GENETICS
Introduction
PROKARYOTES
- Unicellular
- No nucleus
- Structures not surrounded by membrane
- Few internal organelles
EUKARYOTES
- Multicellular
- Nucleus
- Structures surrounded by membrane
- Plant and animal
Cells - The smallest unit of life capable of living in an environment free of life.
​
DNA (Macro-molecule) - Deoxyribonucleic acid: Contains code that determines the shape and structure of living things.
DNA
Nucleotides
Phosphate
Sugar (deoxyribose)
Base
Guanine
Adenine
Cytosine
Thymine
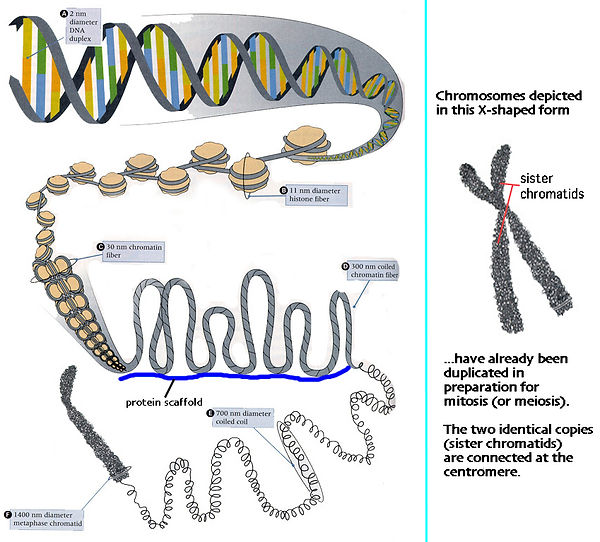
A small segment of DNA carries a gene that stores information used to make a single polypeptide. Polypeptides are used to make proteins and proteins are responsible for determining the characteristics of an organism.
​
Each DNA molecule consists of two strands twisted around each other to form a double helix. A helix is a coiled structure like a corkscrew or spring.
A molecule of DNA is wrapped around proteins to form a single chromatin thread.
​
During cell division, chromatin threads coil tightly into structures called chromosomes inside the cell nucleus.
Adenine pairs with Thymine
Guanine pairs with Cytosine
​
*Order of alphabet determines the genetic code - characteristics of organism.
Figure 1. DNA in chromosomes
The basic unit of DNA is called a nucleotide. Each nucleotide is made up of:
- A sugar called deoxyribose
- A phosphate group
- A nitrogen-containing base
​
- There are four types of nitrogen-containing bases in DNA. The bases are called adenine (A), thymine (T), guanine (G), and cytosine (C).
- Nucelotides can be joined together to form long chains called polynucleotides.
- Each gene is made up of a sequence of nucleotides. The sequences of nucleotides (bases) can vary. This results in many different genes.
- The DNA molecule is made up of two anti-parallel polynucleotide chains.
- According to the rule of base pairing, the bases of one chain are bonded to those of the opposite chain.
​
RULE OF BASE PAIRING
Adenine always pairs with Thymine
Guanine always pairs with Cytosine
(AT & CG)
* Bases are paired together by hydrogen bonds.
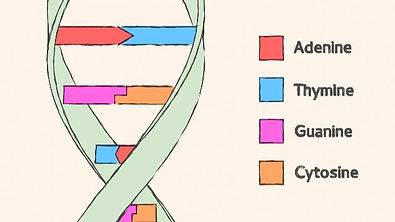
Figure 2. Base Pairings
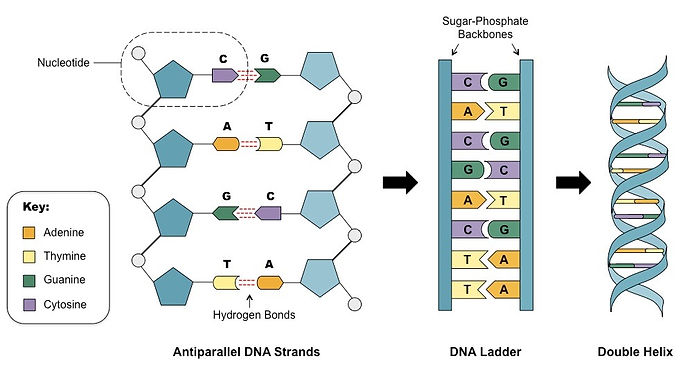
Figure 3. DNA Structure
Transcription & Translation - Making of Polypeptides
In cell division, double helix of DNA becomes single helix and attaches with other single helix.
​
A gene is a sequence of DNA nucleotides that controls the formation of a single polypeptide, which can be used to make proteins. Each gene stores a message that determines how a protein should be made in a cell. This message is known as the genetic code.
RNA - A nucleic acid containing sugar ribose and the base uracil instead of thymine. Unlike DNA it is single stranded / single helix.
​
Steps in making polypeptides:
1. The regions of DNA carrying the gene unwinds and unzips. The two DNA strands are separated.
2. RNA copies the genetic code from the DNA strand through the process of transcription. mRNA (messenger RNA) created.
3. mRNA leaves the nucleus through the nuclear pore
4. mRNA attaches to a ribosome
5. Ribosome translates the message in mRNA into a sequence of amino acids joined together to form a polypeptide. As the ribsosome moves along the mRNA, the polypeptide produced gets longer as more amino acids are joined together by peptide bonds. At the end of the mRNA strand, the ribosome detaches from the mRNA, and the polypeptide is released.
​
The order of amino acids in a polypeptide molecule depends on the order of codons on the mRNA. The order of codons on the mRNA is copied from the complementary DNA template in the nucleus. In other words, the order of bases in DNA molecules forms the genetic code for the formation of a protein.
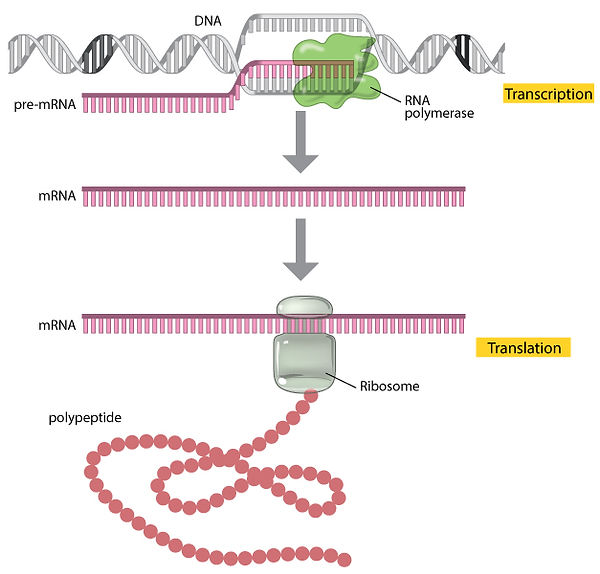
Figure 4. Making of Polypeptides
Mutations
Mutations - Changes
Can be caused by mutagens (e.g. ionizing rays, chemicals, X-rays)
​
TYPES OF MUTATIONS
1. Substitution - Exchange of one base for another
2. Insertion - Extra base pair inserted into a new place in the DNA
3. Deletion - Section of DNA is lost
4. Inversion - Entire segment of bases is inversed
​
Mutations can cause problems in offspring if in the area being copied by the mRNA. Chromosomes being lost or repeated during cell division can also cause problems. Down Syndrome is the result of whole extra chromosome 21 due to faulty cell division in egg/sperm.




Figure 5. Insertion, Substitution & Deletion
Figure 6. Inversion
Genetic Engineering
Genetic Engineering - A technique used to transfer genes from one organism to another. Individual genes may be cut off from the cells of one organism and inserted into the cells of another organism of the same or different species. The transferred gene can express itself in the recipient organism.
A vector is required for the transfer of genes. A vector can be another DNA molecule, a bacterium or a virus that is used to carry the genes of one organism into another. Small circular DNA called plasmids, obtained from bacteria, can be used to transfer genes. A plasmid is an example of a vector.
​
TRANSFERRING HUMAN INSULIN GENE INTO BACTERIA
People suffering from type 1 diabetes usually cannot control the amount of sugar in their blood. Insulin is needed to treat such patients. Insulin used to be obtained from the pancreas of slaughtered animals but animal insulin is not the same as human insulin. Many diabetics develop antibodies against animal insulin after prolonged treatment. Such patients become allergic to animal insulin =, and can no longer use it to control their diabetes. Also, diseases may be transmitted from animals to humans who use animal insulin.
Genetically produced insulin is the same as ordinary human insulin. The advantages of genetically producing insulin are:
- Does not induce allergic response in the patient as the insulin produced is identical to human insulin
- Easier and cheaper to produce insulin in large quantities
- Less risk of contamination by disease-causing microogranisms like bacteria as compared to animal insulin
- The ethical concerns of vegetarians or religious groups can be overcome.
​
Any organism which acquires a foreign gene is called a transgenic organism.
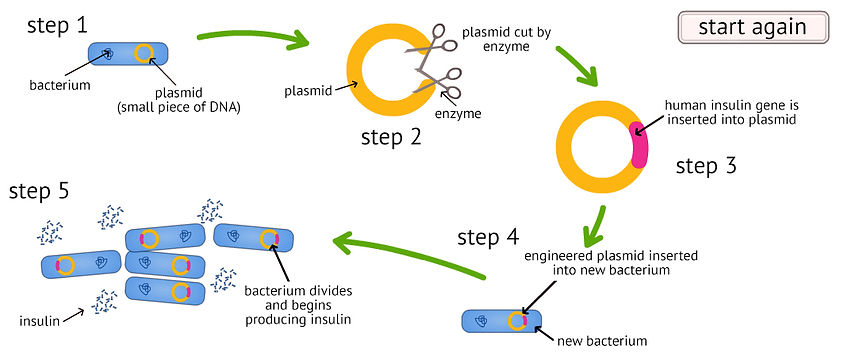
Figure 7. Process of producing human insulin through genetic engineering
PROCESS
1. Obtain the fragment (segment) of DNA in human chromosome that contains the insulin gene. Cut the gene using a restriction enzyme. This enzyme cuts the restriction site at the two ends of the gene to produce 'sticky ends'. Each 'sticky end' is a single strand sequence of DNA bases. These bases can pair with complementary bases to form a double strand.
2. Obtain a plasmid from a bacterium. Cut the plasmid with the same restriction enzyme. This produces 'sticky ends' complementary to the ends of the insulin gene.
3. Mix the plasmid and the DNA fragment containing the human insulin gene. The human insulin gene will bind to the plasmid by complementary base pairing between the sticky ends. Add the enzyme DNA ligase to seal the human insulin gene to the plasmid. This plasmid containing DNA from two different organisms is called recombinant plasmid.
4. Mix the recombinant plasmid with E.coli bacterium. Apply temporary heat or electric shock. This opens up pores in the cell surface membrane of the bacterium for the plasmid to enter.
5. This transgenic bacterium will use the new gene to make insulin. Such bacteria can be isolated and grown for mass production of human insulin. The human insulin has to be extracted and purified before it can be used.
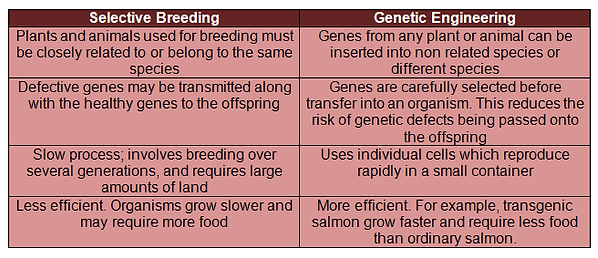

Social & Ethical Implications of Genetic Engineering
Although genetic engineering improves the quality of human life (producing insulin, creating pest-resistant crops), it has also resulted in many social and ethical issues. These are listed below:
1. New proteins in GM (Genetically modified) food might cause allergies in humans that consume them.
2. GM food may prove to be toxic or cancer-causing to people that consume them. Modifying a single gene in plants could result in the alteration of some metabolic processes within the plant. This may result in the production of toxins not usually found within these plants. The consumption of such plants could pose serious health problems.
3. The resulting deaths of useful insects, like the honey bees and butterflies, that feed on the nectar of GM crops may result in a loss of biodiversity.
4. Some biotechnology companies have engineered crop plants such that these plants produce seeds that cannot germinate. This means that farmers have to buy special seeds from the companies annually. This poses a serious problem to poorer societies, where farmers are struggling to make a living.
5. There are objections to consuming animal genes in plants or vice versa. E.g. vegetarians may object to the transfer of animal genes into crop plants.
6. Some people feel that it is morally wrong to exploit animals for medical research, especially when the animals are designed to suffer. E.g. the oncomouse is a transgenic mouse into which a gene that causes cancer has been introduced. The mouse develops cancer for use in testing potential cancer drugs.
7. Some people may deliberately create new combinations of genes which they may use in chemical or biological warfare.
8. Genes that code for antibiotic resistance may be used in genetic engineering. There is concern that such genes might accidentally be incorporated into bacteria that cause diseases to humans, making antibiotics ineffective in treating these diseases.
9. Genetic engineering may lead to class distinction. Only individuals with sufficient financial means can afford certain gene technologies.